
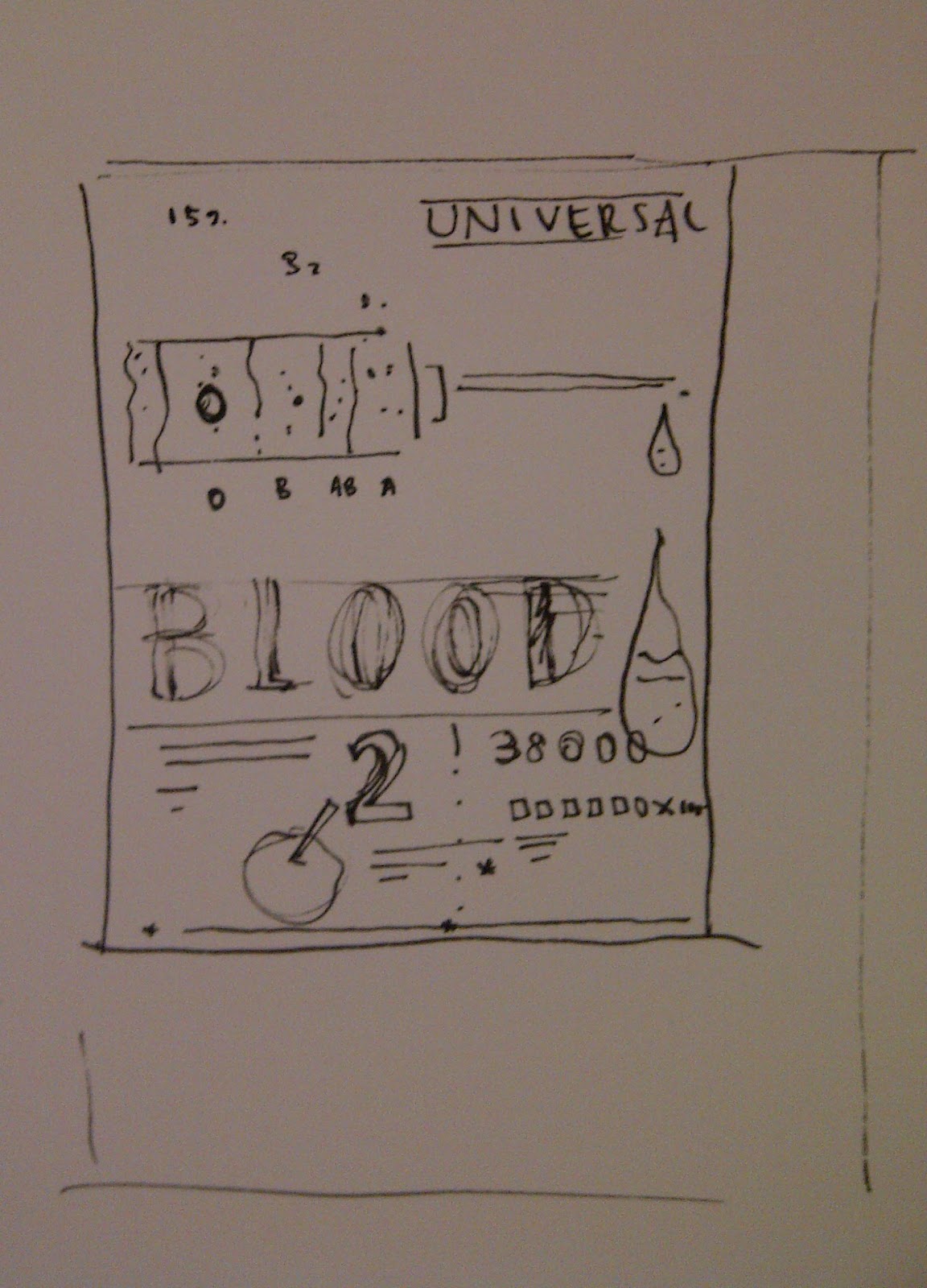
The only exceptions are the so-called nonsecretors for whom a mutation in one of the fucosyl transferases leads to the absence of ABH antigens on secretory epithelial cells in the salivary glands, gastrointestinal tract, and respiratory cavities ( 8). This applies to transfusion of whole blood, RBCs, or platelets as well as tissue or organ transplants, because ABO antigens are not only present on RBCs but also on most other tissues in the human body. Careful matching of the host and donor ABO blood types is essential to avoid transfusion incompatibility events, which are fatal in 10% of all cases ( 7). The A, B, and H (O-type) carbohydrate antigens of the ABO blood group system are the most important clinically ( 3, 5), with about 1 million antigens present on the surface of each RBC ( 6). The majority of the antigens are integrated into the cell membrane, but some, like the Lewis system, are plasma antigens that are adsorbed onto the red cell surface ( 4). These blood group antigens are based either on oligosaccharide epitopes (ABO, P, and Lewis antigens) or on specific amino acid sequences of proteins (Rh, Kell, and Duffy antigens).

#UNIVERSAL BLOOD TYP PLUS#
The human blood group system is complex 30 discrete blood groups are known, defined by 270 antigens plus 38 that have not been assigned to a particular group ( 3). However, problems remain, especially given the challenge of correctly matching blood types on all occasions. This has helped to reduce the usage of blood products, somewhat stabilizing supply versus demand. To counter this demand, significant improvements have been made in the collection and management of blood-component supply, including reassessment of policies concerning when blood is needed and in what quantities. This growth is fueled in part by the demographically aging population and consequent rise in blood-intensive procedures, such as solid-organ transplants, hematopoietic stem-cell transplants, hemorrhage resuscitation, and aggressive chemotherapy against cancer. This requires a massive organization to secure supplies, and yet, according to the World Health Organization, demand for RBCs is expected to grow further ( 2). To make this possible, ∼85 million units of packed red blood cells (RBCs) 2 are obtained annually around the world, according to the Red Cross, largely via donation. We discuss remaining challenges and opportunities for the use of such enzymes in blood conversion and organ transplantation.īlood transfusion is an indispensable part of the health care system, saving many thousands of lives annually. This promising system works well both in standard conditions and in whole blood. In 2019, screening of a metagenomic library derived from the feces of an AB donor enabled discovery of a significantly more efficient two-enzyme system, involving a GalNAc deacetylase and a galactosaminidase, for A conversion. New α-galactosidases and α- N-acetylgalactosaminidases were identified by screening bacterial libraries in 2007, allowing improved conversion of B and the first useful conversions of A-type RBCs, although under constrained conditions. The first demonstration of a B-to-O conversion by Goldstein in 1982 required massive amounts of enzyme but enabled proof-of-principle transfusions without adverse effects in humans. Thus, conversion of A, B, and AB RBCs to O-type RBCs should be achievable by removal of that sugar with an appropriate glycosidase. A and B blood differ from the O type in the presence of an additional sugar antigen (GalNAc and Gal, respectively) on the core H-antigen found on O-type RBCs. This universal donor O-type blood is crucial for emergency situations where time or resources for typing are limited, so it is often in short supply. Of the four main blood types, A, B, AB, and O, only O can be given to any patient. Transfusion of blood, or more commonly red blood cells (RBCs), is integral to health care systems worldwide but requires careful matching of blood types to avoid serious adverse consequences.
